Zoom to microworld - Applications
Thin films
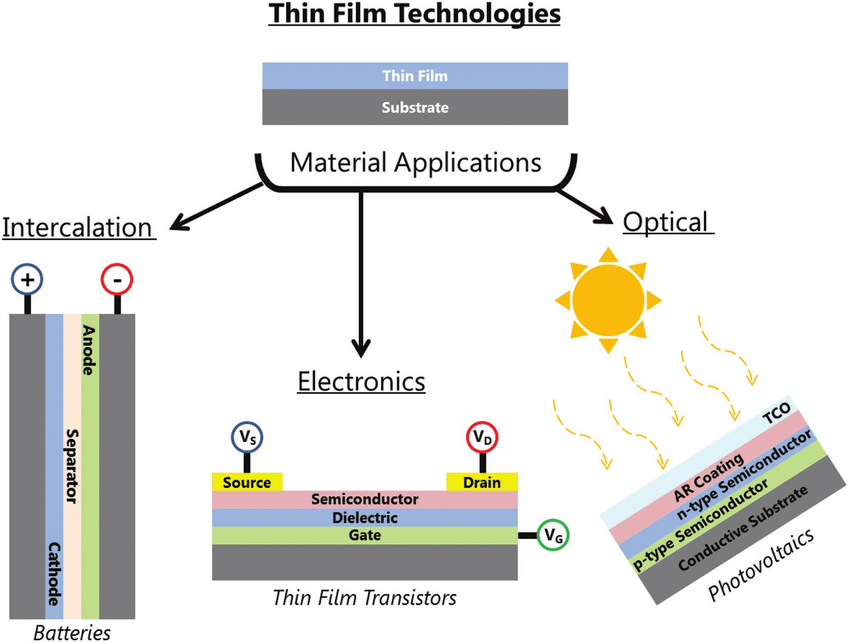
Img src
Hard discs generally use thin films of a Co–Pt alloy. Thin film heads for magnetic recording typically use films of Fe–Ni or Fe–Co alloys in the writer and thin film stacks comprising Fe–Co and Mn-based alloys in the reader. These are soft magnetic films with a good high-frequency response, except for the Mn alloy which is an antiferromagnet. For flexible magnetic recording media, tapes and floppy discs, acicular fine particles of Fe, or Co-doped γ -Fe2O3, are commonly used.
Article src: Magnetism and Magnetic Materials, J. M. D Coey, Cambridge University Press, 2010.
Ferrofluids
The main application area for ferrofluids is in seals. An oil-based fluid is held in place by a suitable magnet, and if oil with a low vapour pressure is chosen, a rotary vacuum seal can be obtained. Applications include bearings for turbopumps and rotary feedthroughs for vacuum systems. Ferrofluid seals around the voice coils of loudspeakers provide damping and a path for dissipating heat. Other uses are as magnetic inks, and in magnetic levitation or magnetic separation.
A different use is made of oil-based dispersions of ferromagnetic particles in magnetorheological fluids. Here the particles are micrometre-size and multidomain, and the loading is much higher, f ∼ 70%. Dipolar forces become strong as the particles are magnetized. The viscosity of the fluid can therefore be increased by orders of magnitude by an applied field. These magnetorheological fluids are used in mechanical clutches and suspension systems. Special ferrofluids are colloidal suspensions of acicular or plate-like ferromagnetic particles in a liquid crystal. It is then possible to influence transitions between various ordered phases in the liquid crystal with a modest magnetic field.
Article src: Magnetism and Magnetic Materials, J. M. D Coey, Cambridge University Press, 2010.
Magnetic microsystems
Miniaturized mechanical drives such as microactuators and micromotors present new opportunities for permanent magnets. This is the field of magnetic MEMS (MicroElectroMechanical Systems). These microdevices have to exert a force on some small object such as a silicon cantilever, so it is important to understand how the forces scale as the dimensions are reduced. We have already seen that the dipole field is invariant as all the dimensions are scaled up or down by a factor ξ.
If there is a conductor in the vicinity of a magnet, the Lorentz force on it per unit volume depends on the vector product of B and current density j. This, too, is scale-independent because jc is an intrinsic property of the conductor. The properties of permanent magnet micromachines based on magnet/current forces depend only on the materials properties Br and jc, where jc is the maximum current loading.
Advantages of MEMS include reduced mass, increased resonant frequencies, lower force constants, and ease of ‘manufacturing’ large numbers of devices simultaneously using silicon (Si) planar processing. MEMS are now seen commercially in applications such as mirrors for optical communications.
One potential application of hard magnetic films is MicroElectroMechanical Systems (MEMS), that are prepared from relatively thick films of the order of several micrometers and the high (BH)max of rare-earth magnets promises small lateral dimensions. Several MEMS components such as a submillimeter electric motor and a stepper motor were successfully prepared. Another application is the creation of a bias magnetic field on the monolithic microwave integrated circuits (MMICs).
Article src: Magnetism and Magnetic Materials, J. M. D Coey, Cambridge University Press, 2010.
Article src: Handbook of Magnetism and Advanced Magnetic Materials, H. Kronmuller and S. Parkin. Volume 1: Fundamentals and Theory, John Wiley & Sons, 2007.
Data storage
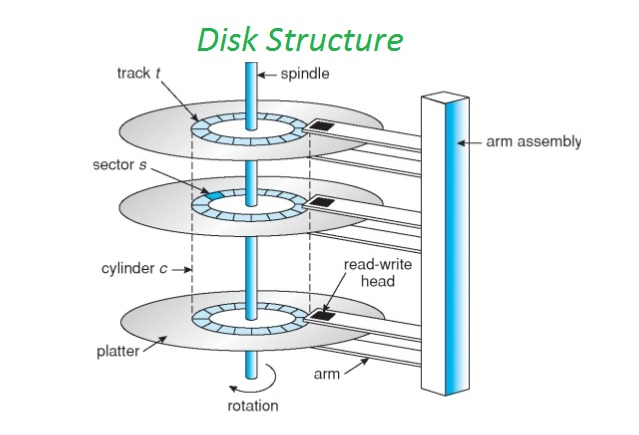
Img src
A very important application of magnetic recording is data storage. Data storage using magnetic tapes is cheap but slow and therefore not suitable for applications where fast access is important. The first data-storage tapes, used in the United States in 1951, had bit lengths of about 200 μm and areal storage densities of the order of 0.002Mb in.−2. Tape storage systems range from ordinary audiocassettes to large reel to- reel systems for mass storage. Audiocassettes were a popular choice for some home computers in the 1980s but are no longer used. By contrast, reel-to-reel and sophisticated cartridge systems continue to be used for purposes such as data backup. For example, present-day tapes have capacities of up to 320 GB.
Diskettes or floppy disks are a very convenient storage medium with moderate capacity. The first floppy disks, introduced in 1971, had a diameter of 8 in. and a storage capacity of 0.08 MB. Early personal computers used 51/4’’ floppy disks, whereas single-sided single-density 51/4’’ disks, first produced in 1976, had a capacity of 0.18 MB. The 31/2’’ disk format was introduced by Sony in 1981. The widely used 31/2’’ DS/HD disks, which date back to 1987, have a storage capacity of 1.44MB and an areal density of more than 2.4Mb in.−2, as compared to about 0.3Mb in.−2 in early 51/4’’ disks (1MB in.−2 = 0.155GB cm−2). The traditional way of producing floppy discs was to start from magnetic thin films that were isotropic in the plane. The disks were then obtained by cutting.
Article src: Handbook of Magnetism and Advanced Magnetic Materials, Helmut KronmÜller and Stuart Parkin. Volume 1: Fundamentals and Theory, John Wiley & Sons, 2007.
Magnetic recording heads
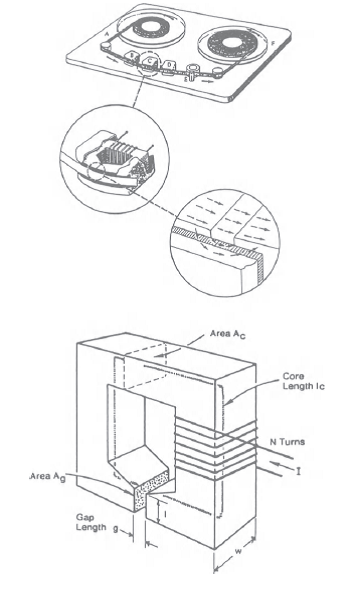
Img src
Recording heads are small electromagnets in the form of a ferromagnetic core with a very small pole gap of typical width 0.3μm. The recording head material must have high saturation magnetization in order to leave a large imprint (high magnetization) on the tape but it must also have low remanence to ensure that there is no writing when the current in the coil is zero. Further it is also clear that a low coercivity is desirable. Recording heads are constructed of magnetically soft material. Many of the soft magnetic materials are suitable for the cores of recording heads; these include soft ferrites, AI-Fe, AI-Fe-Si, Permalloy, and amorphous cobalt-zirconium.
The magnetic tape or disk passes the head where the fringing field causes a realignment of the magnetization within the single-domain articles. The magnetization in the tape is then a record of the strength of the field in the gap of the recording head at the time that the tape passed it. In reading mode the passage of the tape causes a variation in flux density in the reading head which is then converted into a voltage in the coil wound on the reading head. The signal is then amplified and, in the case of audio recording, used to activate a loudspeaker.
Article src: Introduction to Magnetism and Magnetic Materials (1nd edition), David Jiles, Chapman & Hall/CRC, 1991.
Magnetic recording materials
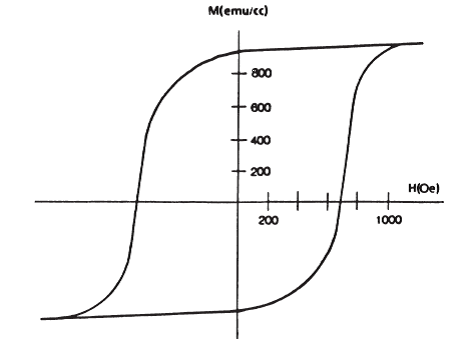
Img src
Magnetic recording materials have some characteristics in common with permanent magnets in that to be useful they need to have a relatively high remanence and a sufficiently high coercivity to prevent unanticipated demagnetization resulting in the loss of information stored on the magnetic tape or disk. Magnetic recording can either be analog, as in audio recording of signals on magnetic tape or digital recording, as used in the storage of information of data on magnetic disks and tapes for computer applications.
The most widely used magnetic recording material is y-Fe203 (gamma ferric oxide) although both chromium dioxide and cobalt-doped y-Fe203 are also used. Gamma ferric oxide is used in both equiaxed and acicular form. Equiaxed gamma ferric oxide particles used for magnetic recording have diameters of 0.05-0.3 μm. Magnetic recording tapes contain small needle-shaped particles of one of these oxides. The particles are embedded in a flexible binding material and at present the needles lie in the plane of the tape. The needle-shaped particles are aligned by a magnetic field during the fabrication process. The final tapes of y-Fe203 have coercivities typically of 20- 24 kA/m, and the acicular particles have lengths ranging from 0.1-0.7μm, with length-to-diameter ratios from 3:1 to 10:1. Tapes made from Cr02 have coercivities of 36-44kA/m. The chromium dioxide particles have dimensions ranging from 0.5 x 0.03μm to 0.2 x 0.02μm which are significantly smaller than the typical sizes of gamma iron oxide particles used in recording tapes. In all cases the ferromagnetic particles used in magnetic recording are too small to contain a domain wall and we therefore have single-domain particles.
Attempts are being made to develop 'perpendicular recording' media in which the needles lie perpendicular to the plane. The advantages of this are that it may be possible to increase the information storage density. Research into perpendicular recording media is continuing, in particular much attention is being directed towards CoCr layers for this purpose. However so far the development of these media has encountered difficulties, among which is the fact that the material does not perform as well as was expected.
The hysteresis loops which are desirable for magnetic recording materials are generally square loops, with high remanence, moderately high coercivity and rapid switching from one state to the other, as shown in figure, which is the hysteresis loop of a metallic magnetic recording medium. In this case the coercivity 56 kA/m and remanence 0.9 x 106 A/m are substantially higher than for y-Fe203 particles.
Article src: Introduction to Magnetism and Magnetic Materials (1nd edition), David Jiles, Chapman & Hall/CRC, 1991.
Magnetic bit

Img src
A key figure of merit of magnetic recording media is the areal storage density, measured in bits per square centimeter (b cm−2) or bits per square inch (1 b in.−2 = 6.452 b cm−2). Figure shows schematically the development of the areal storage density in recent decades. Since its invention, magnetic recording has developed rapidly, competing with and often outperforming other storage media, from vinyl records and punch cards to advanced electronic and optical storage media such as CD-ROMs and flash memory. The main advantages of magnetic storage are the potential density and cost. The bit size of optical and magneto-optical media is limited by the wavelength of the used light, whereas semiconductor devices can be slow and/or volatile.
Defining an effective bit size as the square root of the inverse areal density, the progress shown in Figure corresponds to a bit-size reduction from 250 μm in 1960 to 250 nm in 2000. The recent trend is likely to continue in the next few years, with terabit recording at the horizon. An upper limit to the recording density is provided by the thermal stability of the stored information, which makes it difficult to realize room-temperature magnetic recording using bit sizes smaller than several nanometers.
Article src: Handbook of Magnetism and Advanced Magnetic Materials, H. Kronmuller and S. Parkin. Volume 1: Fundamentals and Theory, John Wiley & Sons, 2007.